What is Multiple Myeloma?
Multiple myeloma (MM) is a cancer of the blood that develops in the plasma cells in bone marrow. Plasma cells assist the immune system by producing antibody proteins as a response to bacterial infections. When cancer grows in these cells it causes abnormal plasma cell growth and leads to the formation of malignant tumours throughout the bone marrow.1 Symptoms of MM generally do not develop until the cancer has advanced and spread.2 It is also a heterogeneous disease, which means there is no one-size-fits-all method of treatment. This implies that personalized risk stratification is essential for disease treatment and management.3
The American Cancer Society estimates that about 34,470 new cases of multiple myeloma will be diagnosed in 2022—19,100 in men and 15,370 in women.4 Prognostic and predictive biomarkers for multiple myeloma are heavily being investigated as a way to monitor cancer treatment progression and as a way to categorize people that are more likely to respond to a treatment.

The Search for Multi-Panel Biomarkers and Risk Stratification of MM
Researchers within the field of oncology know that biomarker discovery is a world where precision and specificity is essential. For a successful prognostic/diagnostic test to be brought to the market, it needs to have a high level of specificity to the cancer that it is screening for. However, there is increasing evidence that various stages of cancer progression will exhibit different biomarkers. This means that one biomarker is not a strong enough tool for the molecular characterization of cancer signatures. As a result, multi-biomarker panel signatures are increasingly being studied for risk-stratification to predict patient survival and as predictive markers for treatment response. A 2019 literature review by Lê et al. found that the use of large panels of biomarkers are less likely to yield unreliable results owing to the substantial heterogeneity among cancers.3
NORBLOG
Want to hear more from Norgen?
Join over 10,000 scientists, bioinformaticians, and researchers who receive our exclusive deals, industry updates, and more, directly to their inbox.
For a limited time, subscribe and SAVE 10% on your next purchase!
SIGN UP
All Eyes on Exosomes - A Cargo of Information
Exosomes continue to predominate the nano world as a treasure chest of information, particularly when it comes to disease diagnostics. They are small (40 nm - 150 nm) membrane-bound vesicles produced by all living cells and can be found in all body fluids, including but not limited to plasma, urine, saliva, breast milk, amniotic fluids and others.
Among their numerous functions, they are involved in conveying cellular information to distant cells and tissues within the body, where they can alter function and/or physiology. Exosomes act as natural carriers of microRNA and can also contain distinct subsets of microRNAs which depend upon the tumour cell type from which they are secreted (tumour-specific signatures). A recent review of next-generation biomarkers in MM presented promising research on how circulatory exosomal miRNAs such as let-7b and miR-18a possess a prognostic role in MM by predicting the progression of the disease.5
Tumour-Specific Content in Exosomes
According to a recent 2021 study published in Frontiers in Medicine, Rezaei et al. determined that the average size of tumor-derived exosomes to be 95 nm.6 They performed analyses on tumor-derived exosomes (TEXs) using a Zetasizer machine, which performs size measurement analysis using a process called Dynamic Light Scattering and enables measurements of particles and molecules on the nanoscale.7 Other sources in the literature describe these exosomes as measuring less than 50nm in diameter.8 Confirming the presence and size of these particles is but one part of the puzzle to exploring their role and mechanism within a disease state. The challenge exists in its isolation and purification for further downstream analysis.
Exosome Purification - Is There a Better Way?
Although ultracentrifugation (UC) is the most widely used method of EV purification, there are critical limitations reported among literature, some of which include co-isolation of non-exosomal contaminants, potential damage of exosomes and low-throughput of samples.9 A comparison of traditional and novel methods for the separation of exosomes from human samples found that ultracentrifugation may result in a large number of exosomes being lost at the risk of reducing the yield.10
Promising research published in Frontiers of Oncology (2020) identifies how “circulating exosomal miRNAs isolated from the serum of 156 patients identified 22 miRNAs expressed at a significantly lower level in MM patients compared to healthy individuals... among those, let-7b and miR18a were significantly associated with both progression-free survival and overall survival.”11 This exciting work adds to the notion that exosomes may be a useful marker of disease progression for multiple myeloma.
Resin-Based Isolation of Small Plasma/Serum EVs
In 2021, Australian researchers investigated the biological activity and characterization of plasma-derived extracellular vesicles (psEV) from human multiple myeloma cell lines (HMCL) in order to explore their potential as oncogenic biomarkers in myeloma disease.1
The isolation of psEV from blood remains an arduous task due to the presence of highly abundant proteins and other contaminants which can co-isolate and impair the sample. Since the recovery of psEV is highly dependant on the method of isolation, the scientists decided to compare the performance of three commercially available exosome purification kits and develop a method to study the biological mechanisms of psEV within the context of MM.1
The team investigated the efficacy of several commercial kits on the isolation of plasma/serum EV from frozen plasma. They compared a precipitation method (ExoQuick ULTRA), a membrane-based affinity method (exoEasy) and a resin-based capture/enrichment method (Norgen). The results below (Figure 3C) show that in comparison to Exoquick and ExoEasy purification methods, the resin-based method provided the cleanest exosomes with high purity and minimal background contamination. Exoquick and Exoeasy purification reflects co-isolation of abundant plasma proteins as can be seen with the high TEM background.
It is interesting to note that a 2018 publication that compared the exosome purification between exoEasy and size-exclusion chromatography found that the exoEasy kit isolated a heterogenous mixture of particles with larger median diameter and a broader size range.12 It also noted the presence of contaminating proteins (albumin) and very low levels of exosome-associated proteins.12
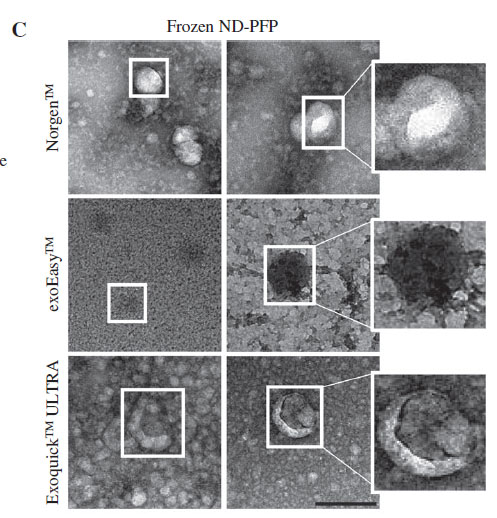
The precipitation method (Exoquick) on the other hand, requires a thrombin pre-treatment for compatibility with its precipitation buffer. Recent literature in the Journal of Circulating Biomarkers found that thrombin reduces the recovery of extracellular vesicles from blood plasma and should be omitted from the protocols, describing how thrombin triggers clot formation which may entrap many EVs and lead to exosome loss.13 The resin-based kit requires no pre-treatments, filters or size exclusion to capture EVs; instead, it operates on the pH of the exosomal surface proteins and other specialized buffers in a capture and release method using the silicon carbide resin. The researchers found that the resin-based kit provided the highest enrichment of exosomal protein markers when compared to the precipitation and membrane-based affinity methods.
Comparing Exosome Quality: Fresh vs. Frozen Plasma
In addition to confirming which kit purified the cleanest exosomes, the team also went on to investigate if there was a difference between exosomes purified from fresh versus frozen plasma. Reale et al. (2021) performed a western blot analysis on the plasma serum EV isolated with the resin-based kit using EV markers (TSG101, CD81, ALIX, CD63) and platelet markers (ITGA2B and SELP) (Fig 2). They discovered that the bands of the protein markers were much more distinct in the fresh samples than in the frozen samples. This implies that although exosomes can be purified from both frozen and fresh plasma, fresh plasma samples yield higher quantities of EVs. It was also demonstrated that fresh samples displayed a higher EV protein profile (TSG101, CD81, ALIX, CD63) compared to the frozen samples (Fig.2).
A study from PLOS ONE (2021) also made similar conclusions, finding that the plasma-derived EV concentration of stored samples decreased to 23% of fresh samples.14 One reason to explain this difference is that the lipid bilayer of samples frozen at -80˚C may be exposed to a variety of physical stressors, resulting in damage to the cellular components and ultimately affecting EV yield and it's molecular content.
The team also measured the extent of platelet-derived EVs using platelet markers TGA2B and SELP, and found them to exhibit minimal contamination. This data corroborates what is found in much of the literature, which is that EV yield in plasma/serum is strongly influenced by storage conditions, and fresh samples retain more EVs than frozen samples.
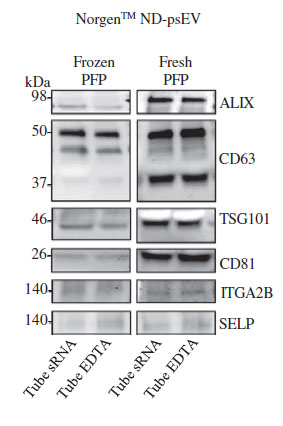
Future Implications of Plasma/Serum Biomarkers
This work demonstrates the need for standardization of pre-analytical factors within sample preparation and careful selection of EV purification methodologies within the field of oncological research. It provides optimistic insights into future studies of multiple myeloma and the potential of plasma/serum biomarkers within clinical and diagnostic settings.